In PROfound, 69% (2792/4047) of patients who underwent tissue testing had an NGS result. While NGS result generation was possible for all tissue sample types (ie archival vs newly collected and primary vs metastatic sites), pre-analytical factors including sample age, tumor content and DNA yield impacted likelihood of obtaining an NGS result (determined from observed and modeled data). Optimization of tumor tissue collection, preservation, and processing to provide high-quality samples for genomic testing has the potential to identify patients with mCRPC who are eligible for targeted treatments, such as olaparib.

PROfound (NCT02987543) is the largest Phase III poly(ADP-ribose) polymerase (PARP) inhibitor study to date to conduct central, prospective tissue next-generation sequencing (NGS) to screen patients with metastatic castration-resistant prostate cancer (mCRPC) to determine eligibility for enrollment.1,2 Patients were required to have a qualifying alteration in one or more of 15 pre-specified deleterious or suspected deleterious germline or somatic homologous recombination repair (HRR) genes (ATM, BRCA1, BRCA2, BRIP1, BARD1, CDK12, CHEK1, CHEK2, FANCL, PALB2, PPP2R2A, RAD51B, RAD51C, RAD51D, RAD54L). Tumor testing is the gold standard for molecular testing, but ensuring that it is routinely used to identify patients with mCRPC who have qualifying alterations for PARP inhibitor treatment is a challenge.
Evaluation of tumor testing outcome against pre-analytical parameters from >4000 patient samples in PROfound identified key factors influencing NGS result generation. Although most tissue samples were from archival tissue and primary prostate tumor specimens (see organ breakdown in Figure 1), the rate of NGS results generated was marginally higher in more recently obtained and metastatic samples (both 63.9%) than archival (56.9%) and primary prostate samples (56.2%); however, generation of an NGS result was possible with all sample types. Differences in rates of obtaining an NGS result across organ types were noted (Figure 2A). There was a decline in the rate of NGS results with increased sample age, which may be a result of DNA degradation over time; however, almost half of samples aged >10 years, especially those with larger volume or high tumor content, had an NGS result suggesting that optimizing collection methods may help with obtaining NGS results from archival samples (Figure 2B). As may be expected, there was an increase in the proportion of NGS results obtained with increased tumor content and DNA yield (Figure 2C & D).
Figure 1. The proportion of specimen tissue type from 4858 tumor samples (taken from 4047 patients with samples eligible for assessment)
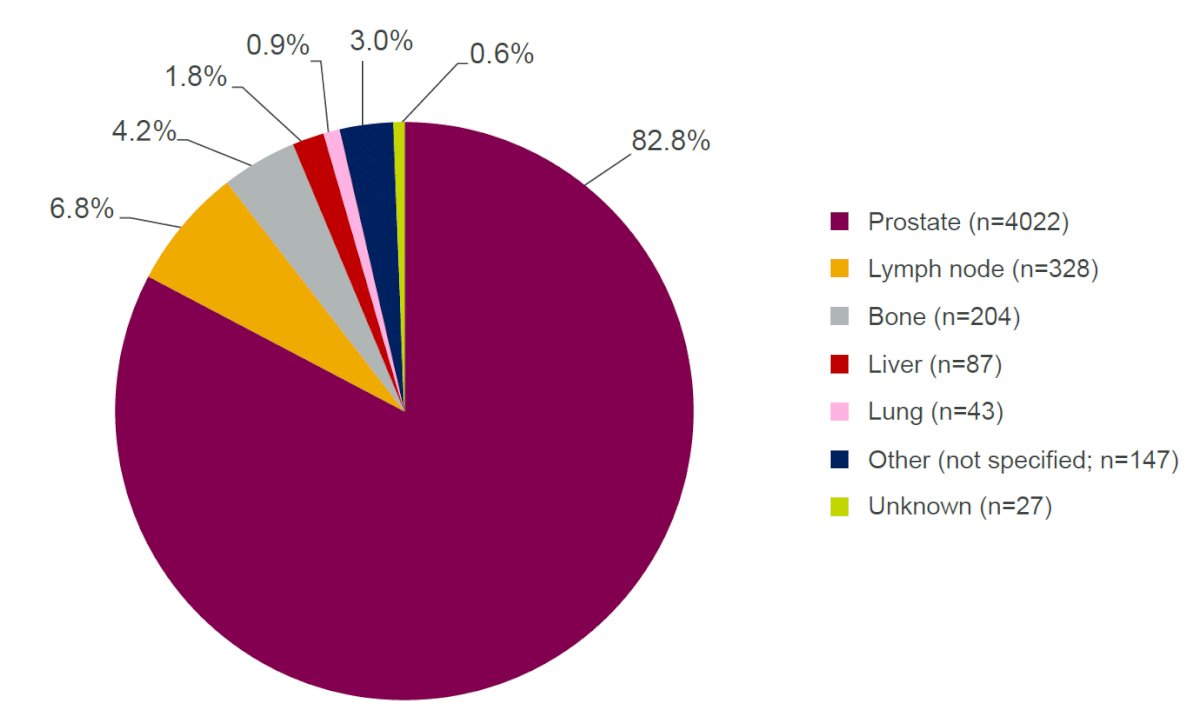
Figure 2. Proportion of samples for which a next-generation sequencing (NGS) result was generated by (A) organ site; (B) sample age; (C) tumor content; and (D) DNA yield
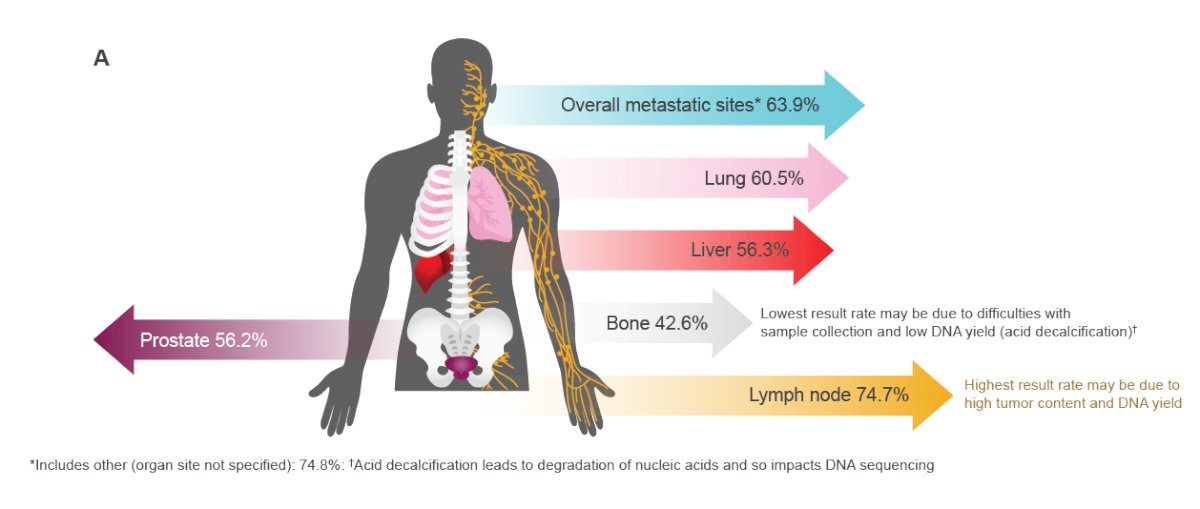

Core needle biopsy (CNB) is increasingly being used to obtain prostate tissue samples as it is less invasive than many other collection methods, and it was used for almost two-thirds of samples in our study; however, NGS result rates were lower (52.4%) than for other collection methods (eg 74.0% for radical prostatectomy, 69.8% for transurethral resection of the prostate and 61.5% for excisional biopsy). This may be due to the CNB samples not meeting adequate specimen volume and percentage tumor nuclei requirements. However, the generation of an NGS result from CNB samples is achievable if several pre-analytical factors are considered, including selection of the most recently collected sample with highest tumor content, embedding multiple CNBs into a single formalin-fixed, paraffin-embedded block, and macrodissection of the tumor area (if the overall volume and cellularity of the sample is sufficient). Improvements in tissue collection methods, such as magnetic resonance imaging (MRI)-guided biopsies, are also likely to ensure that samples with higher tumor cellularity (potentially resulting in higher tumor DNA yield) are collected, which will ultimately improve NGS results for CNBs.3
To improve genomic testing rates, evaluation of reasons for sample failure is also critical. The main reasons for samples failing to meet standard requirements for the test process, and consequently not obtaining an NGS test result, were failure at pathology review (minimum tumor content of 20% and minimum tissue volume of 0.2 mm3 not met) and failed DNA extraction. Archival samples were most likely to fail at the DNA extraction stage, whereas recently obtained samples were more likely to fail at the pathology review stage, primarily due to insufficient tumor content/purity and insufficient tissue size.
Overall, the three factors that had the biggest impact on obtaining an NGS result in PROfound (both in actual data analysis and logistic regression modeling of data from samples that passed pathology adequacy review) were:
- Sample age
- Tumor content
- DNA yield

CNB, core needle biopsies; EDTA, ethylenediaminetetraacetic acid; FFPE, formalin-foxed paraffin-embedded; MRI, magnetic resonance imaging.
Acknowledgments:
This study was funded by AstraZeneca and is part of an alliance between AstraZeneca and Merck Sharp & Dohme Corp, a subsidiary of Merck & Co., Inc., Kenilworth, NJ, USA. Medical writing assistance was provided by Juliet Fawcett, PhD, for Mudskipper Business Ltd, funded by AstraZeneca and Merck Sharp & Dohme Corp., a subsidiary of Merck & Co., Inc., Kenilworth, NJ, USA.
Written by: Maha Hussain,1 Claire Corcoran,2 Caroline Sibilla,2 Karim Fizazi,3 Fred Saad,4 Neal Shore,5 Shahneen Sandhu,6 Joaquin Mateo,7 David Olmos,8 Niven Mehra,9 Michael P. Kolinsky,10 Guilhem Roubaud,11 Mustafa Ӧzgüroǧlu,12 Nobuaki Matsubara,13 Craig Gedye,14 Young Deuk Choi,15 Charles Padua,16 Alexander Kohlmann,17 Robert Huisden,2 Julia A. Elvin,18 Jinyu Kang,17 Carrie A. Adelman,2 Allison Allen,2 Christian Poehlein,19 Johann de Bono20
- Robert H Lurie Comprehensive Cancer Center, Northwestern University Feinberg School of Medicine, Chicago, IL, USA
- AstraZeneca, Cambridge, UK
- Institut Gustave Roussy, University of Paris Sud, Villejuif, France
- Centre Hospitalier de l’Université de Montréal/CRCHUM, Montreal, QC, Canada
- Carolina Urologic Research Center, Myrtle Beach, SC, USA
- Peter MacCallum Cancer Centre and the University of Melbourne, Melbourne, VIC, Australia
- Vall d’Hebron Institute of Oncology and Vall d’Hebron University Hospital, Barcelona, Spain
- Spanish National Cancer Research Centre (CNIO), Madrid, and Instituto de Investigación Biomédica de Málaga (IBIMA), Malaga, Spain
- Radboud University Medical Center, Nijmegen, The Netherlands
- Cross Cancer Institute and University of Alberta, Edmonton, AB, Canada
- Institut Bergonié, Bordeaux, France
- Istanbul University-Cerrahpaşa, Cerrahpaşa School of Medicine, Istanbul, Turkey
- National Cancer Center Hospital East, Chiba, Japan
- Calvary Mater Newcastle, Waratah, NSW, Australia
- Yonsei University College of Medicine, Seoul, South Korea
- Cetus Medicina Oncológica, Betim, Brazil
- AstraZeneca, Gaithersburg, MD, USA
- Foundation Medicine, Inc., Cambridge, MA, USA
- Merck & Co., Inc., Kenilworth, NJ, USA
- The Institute of Cancer Research and Royal Marsden Hospital, London, UK
References:
- de Bono J, Mateo J, Fizazi K et al. Olaparib for metastatic castration-resistant prostate cancer. N Engl J Med 2020;382:2091-102.
- Hussain M, Mateo J, Fizazi K et al. Survival with olaparib in metastatic castration-resistant prostate cancer. N Engl J Med 2020;383:2345-57.
- Schoots IG, Roobol MJ, Nieboer D et al. Magnetic resonance imaging-targeted biopsy may enhance the diagnostic accuracy of significant prostate cancer detection compared to standard transrectal ultrasound-guided biopsy: a systematic review and meta-analysis. Eur Urol 2015;68:438-50.
- Gonzalez D, Mateo J, Stenzinger A et al. Practical considerations for optimising homologous recombination repair mutation testing in patients with metastatic prostate cancer. J Pathol Clin Res 2021;7:311-25.
- FDA. LYNPARZA (olaparib) prescribing information 2020. Available at: https://www.accessdata.fda.gov/drugsatfda_docs/label/2020/208558s014lbl.pdf (accessed 22 December 2020).
- EMA. LYNPARZA (olaparib) summary of product characteristics. 2020. Available at: https://www.ema.europa.eu/en/documents/product-information/lynparza-epar-product-information_en.pdf (accessed 21 December 2020).
Read the Abstract